Plumbing the Depths of Multiphoton Microscopy
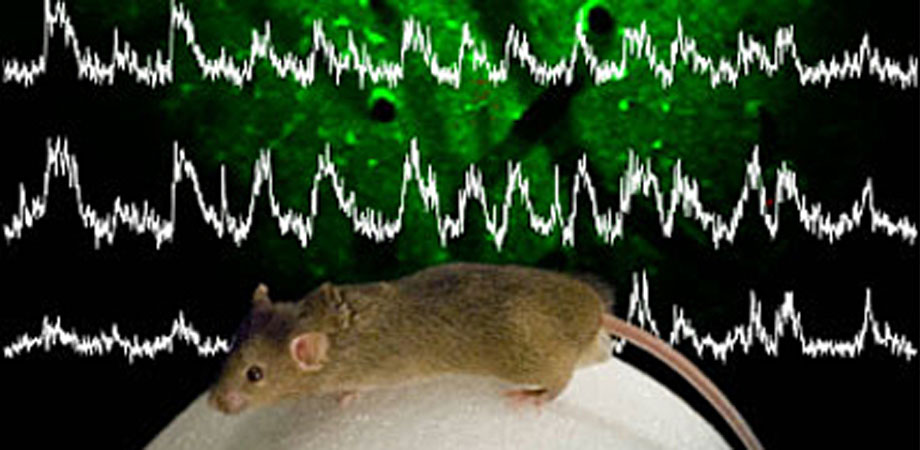
It started as a side project. In fact, when physicist Winfried Denk co-invented multiphoton fluorescence microscopy in 1990, he was transitioning between jobs. Having just wrapped up his PhD at Cornell University and poised to start work as a postdoc in Switzerland, Denk picked up a library book about laser scanning microscopy.
In the book, Denk read about nonlinear optical effects, the interactions between photons and matter that occur when the light source is extremely intense. At the time, Denk's colleagues at Cornell, who studied calcium ions inside biological samples, lacked robust ultraviolet lasers and lenses to image the ions' dynamics. It occurred to Denk that if researchers used a nonlinear effect called two-photon absorption, they could side-step the need for UV optics. Instead of using a single ultraviolet photon to excite a labeled calcium ion to emit light, two visible photons at half the wavelength could cause the same fluorescence.
So Denk tried it. Working with his PhD adviser Watt Webb and Cornell graduate student James Strickler, Denk borrowed a colleague's laser, and they set up the experiment. It took them about six hours to get everything running, and two or three days to take the data they would publish in their landmark 1990 Science paper. "It was not particularly technically difficult," says Denk. In the paper, the team published images of latex bead cross-sections and chromosomes in pig kidney cells.
As the 30th anniversary of its invention approaches, the technique known as multiphoton microscopy has become an indispensable tool for the 3D imaging of thick, living tissue. With the invention of the titanium sapphire laser, a robust light source that did not require laser specialists to operate, the technique took off. Biologists have used multiphoton microscopy to study living tissue and live animals, and some researchers have even begun to consider it for clinical use in dermatology, such as for skin cancer diagnosis. Researchers presented on some new applications of the technique, as well as developments in laser technology, at Sunday's session on Multiphoton Microscopy in the Biomedical Sciences at SPIE Photonics West.
In particular, multiphoton microscopy has become the workhorse of neuroscientists, enabling them to peer deeper into the cellular structure of the brain than ever before. "The biggest application field is neuroscience, the brain," says physicist Chris Xu of Cornell University. "Multiphoton microscopy really shines when you need high spatial resolution-single cell and subcellular imaging-and you have to look at it in intact tissue."
Compared to its parent technique, conventional fluorescence microscopy, multiphoton microscopy captures clearer images at greater tissue depths while reducing photodamage. These advantages arise from the physics. In both techniques, a focused laser beam illuminates the tissue to excite fluorescent molecules. However, instead of using visible light and shorter wavelengths for imaging, in multiphoton microscopy researchers commonly use infrared light, two photons of which collectively cause a fluorophore to fluoresce in the visible. Tissue is more transparent to infrared light versus visible light, which means the photons can image deeper into the tissue, beyond a millimeter.
In addition, two-photon absorption only occurs at the focus of the laser, because only there is the light intense enough to cause two-photon absorption. Thus, fluorescence is confined to a small point, so its detection allows the formation of a high-resolution image. Another advantage is that multiphoton microscopy requires relatively simple optics. It does not even need a camera, just a light detector. Because the fluorescence is confined to such a small area, the researcher simply has to measure the intensity. Software can then calculate the origin of the fluorescence to piece together a larger picture.
Over three decades, the technology has become much more accessible. Denk's original setup involved a confocal microscope and a borrowed laser. "It was one of those cute physics tricks that one uses to impress the biologists," says Denk. "‘Look what I can do for you. It's expensive but you can't do any other way.'"
Now, biologists can use them on their own. Companies such as Nikon, Zeiss, and Olympus offer commercial versions of the technology. Non-specialists can even build a microscope themselves: in 2014, Grant Gordon, a neuroscientist at the University of Calgary, published an open-source design for a two-photon microscope made almost completely of off-the-shelf materials. Gordon learned the basics of the technique at a workshop in Cold Spring Harbor and built his machine over the course of a year.
"Two-photon microscopes are amenable to making yourself," says Gordon. "It has to do with the precision of the light path, which does not have to be extremely accurate." Excluding the laser source, his microscope costs about $70,000, about half the price of a commercial version. Grant uses his to study astrocytes, star-shaped glial cells in rodent brains. To create images, his team uses open-source software offered by researchers at the Howard Hughes Medical Institute. Since Gordon published his design, he knows of around ten labs that have used his design to build their own two-photon microscopes.
The technique, notably called "multiphoton microscopy," is not limited to two-photon fluorescence. In 1996, while working as a PhD student in Webb's lab at Cornell, Xu demonstrated three-photon microscopy, in which a molecule absorbs three photons to fluoresce. "In some sense, three-photon was very natural," says Xu. "If two-photon works, then you add another photon."
The potential advantages of three-photon microscopy were not immediately obvious. After more than a decade of other projects, including a stint in the telecom industry, Xu picked up its development around 2009 at Cornell. Three-photon microscopy, it turns out, can image even deeper than two-photon microscopy. This is in part because three-photon microscopy uses even longer wavelengths of infrared light, around 1.3 and 1.7 microns. In addition, three-photon absorption occurs in even a smaller confined point, leading to an even clearer image. But to develop the method, Xu had to work with the laser industry to invent a robust light source at the right wavelength.
In 2014, Xu's group demonstrated they could perform four-photon fluorescence microscopy, although it has no clear advantage over three photons, especially because water molecules absorb its working wavelengths. Instead, Xu is focusing on further developing three-photon microscopy. The current record for depth is about 2.2 millimeters, he says. "I think we could potentially go in the mouse brain around 2.5 millimeters," says Xu. "That might be the limit."
Sophia Chen contributes to Wired, Science, and Physics Girl. She is a freelance science writer based in Tucson, Arizona.
Related SPIE content:
Faster, wider, deeper: imaging advances in focus at Hot Topics session
Chris Xu: 3-photon microscopy for deep brain imaging
€1m Brain Prize for developers of two-photon microscopy
Enjoy this article? Get similar news in your inbox |
|