Small satellites, big dreams
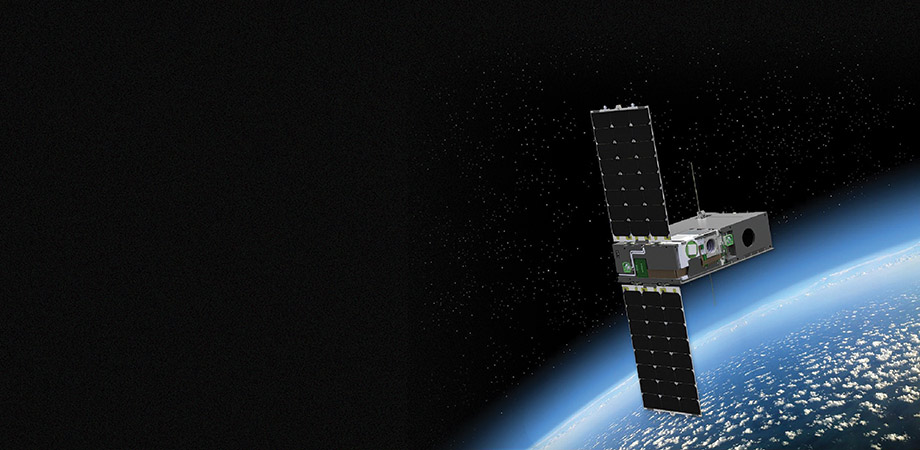
On 30 July 2020, a toaster-sized satellite was ejected from the International Space Station. The cubesat—called DeMi, short for the Deformable Mirror Demonstration Mission—had a tiny mirror the size of a postage stamp. Despite its small size, mission scientists and operators from Massachusetts Institute of Technology (MIT), had big aspirations for their spacecraft. They hoped it would blaze a path toward imaging the first Earthlike planets orbiting other stars.
Such images are exceedingly difficult to obtain with any technology. Of the more than 4,000 exoplanets discovered in the Milky Way, most are known only through indirect signatures, such as with radial velocity and transit photometry methods. Only a few dozen have been imaged directly, mostly the so-called gas giant planets that are far larger than any planet in our solar system.
Detecting, much less imaging, Earth-sized exoplanets, particularly those orbiting Sunlike stars, is nearly impossible with today’s telescopes. Ground-based observatories must overcome limited nighttime observing and combat the distorting effects of the atmosphere, whereas space telescopes do not yet have the time or capabilities to discover other Earths in Earth-size orbits.
Given these challenges, “finding an Earth twin—a planet that’s the size of the Earth, orbiting a star like the Sun approximately at the same distance—would be a big deal,” says Mary Knapp, an astronomer at MIT’s Haystack Observatory. “The existing survey instruments, like Kepler and TESS, they’re just not built for that.”
Astronomers hope next-generation space telescopes will be able to image other Earths and take measurements necessary to determine their habitability. But to get there, new technologies are needed. So, some groups, like the DeMi team, are looking to cubesats—small satellites built out of standardized cubes with 10-cm-long sides.
The DeMi cubesat being ejected from the International Space Station.
First developed in 1999 as an educational tool for universities, cubesats have received increased attention in the past decade primarily due to commercial interests, including for remote sensing, communications, and technology testing. However, their use in science is also increasing. They are relatively cheap, with costs that run a few million dollars instead of billions for some space telescopes. Cubesats offer greater access to space, including by universities and other research institutions, to gather new observations and test innovative technologies.
“Cubesats fit in a sweet spot of cost and efficiency,” says Ewan Douglas, an astronomer and assistant professor at the University of Arizona and payload engineer and project scientist for DeMi. “It’s a very plug-and-play interface because it’s a standard platform.”
This standardization makes cubesats easy to launch and allows for commercial off-the-shelf parts instead of custom-designed components. Turnaround times are also typically a fast five to 10 years from build to launch—much faster than the decades required for larger space telescopes.
In line with their educational origins, cubesats today are launching scientific and engineering careers because university students can gain first-hand experience working on noncrewed space missions.
“With cubesats we’re actually doing cutting-edge science, but still, there’s that strong educational component,” Douglas says.
In recent years, astronomers and engineers have developed new technologies to better image exoplanets from ground-based observatories. To compensate for distortions of light by Earth’s atmosphere, researchers have turned to adaptive optics to make real-time corrections.
Adaptive optics for telescopes use two main components: a wavefront sensor and a deformable mirror. The wavefront sensor detects the shape of the incoming light and feeds that information to the mirror. Under the mirror, tiny pistons called actuators slightly adjust the shape of the mirror’s surface, thus canceling any distortions of the incoming light.
When paired with a starlight-blocking instrument called a coronagraph, adaptive optics have proven successful for ground-based exoplanet imagers. The Gemini South Telescope, the Subaru Coronagraphic Extreme Adaptive Optics on the Subaru Telescope, and the Spectro-Polarimetric High-contrast Exoplanet Research instrument at the Very Large Telescope, have all used such systems to image exoplanets.
And now DeMi, funded by US Defense Advanced Research Projects Agency and managed by private-sector company Aurora Flight Sciences, is demonstrating for the first time in orbit an imaging system with a deformable mirror driven by a microelectromechanical system.
Although there are no atmospheric disturbances of light to contend with, “once you go into space, there’s still enough manufacturing defects and distortion that you need to actively correct the surface of the optics,” Douglas says.
Using adaptive optics in space can harvest big returns. Even a small telescope equipped with adaptive optics to correct for defects and thermal distortions can provide enough imaging improvement to see planets impossible to find with ground-based observatories. The National Aeronautics and Space Administration’s upcoming Nancy Grace Roman Space Telescope will implement adaptive optics, though using a slightly different style of deformable mirror than that onboard DeMi.
The DeMi cubesat awaiting installation into the International Space Station deployer arm. Credit: Nanoracks
DeMi’s deformable mirror uses 140 actuators—as many as could fit within the confines of a cubesat and enough for testing the adaptive optics system, but far fewer than the thousands that would ultimately be needed on an exo-Earth-searching telescope. So far, DeMi’s operators have successfully demonstrated its adaptive optics system in space using a laser diode to simulate a star for the wavefront sensor to detect, and they are testing the system on actual stars.
“DeMi is demonstrating nanometer level control of the optics, which is much better than we can do on the ground,” Douglas says. “But we’ll need to get into the subnanometer, even picometer regime, across much bigger telescopes for these Earthlike exoplanet missions.”
For adaptive optics to work effectively when using a coronagraph, a sufficiently bright star must be in the telescope’s field of view. This light is needed for the feedback system to work fast enough to make its measurements. In cases where such a bright star is not visible, ground-based observatories have used artificial alternatives called laser guide stars.
Laser guide stars are created by shining a laser into the atmosphere where it backscatters or causes a slight glow that can then be used by the wavefront sensor. For future space telescopes using adaptive optics, Douglas and his collaborators, led by Kerri Cahoy at MIT, have proposed using cubesats themselves as laser guide stars. A single cubesat flown with a major mission could help provide a bright reference point allowing for faster and more precise measurement of the telescope’s optical alignment. Such a cubesat could be built with technology in use for space laser communications.
Telescope alignment and pointing can also be disrupted by external factors such as atmospheric drag forces, torque caused by magnetic fields, and radiation pressure from sunlight, all of which can cause a telescope to jiggle. These effects need to be accounted for even when using other detection techniques like transit photometry, which watch for a slight dip in light coming from a star as a planet crosses its face.
“All of these things push your telescope around a little bit,” Knapp says. “When that happens, the star moves a little bit on the detector, and you can get a false signal.”
In recent years, Knapp has worked as project scientist with a cubesat mission called ASTERIA (Arcsecond Space Telescope Enabling Research in Astrophysics) that’s trying to resolve these challenges. Deployed in 2017, the ASTERIA cubesat primarily tested a high-precision pointing technology that could improve exoplanet detections by transits, which is by far the most common way of finding exoplanets.
“It’s kind of like a Steadicam,” Knapp says, referring to the camera stabilizer mounts used for videography. “It’s meant to compensate for those disturbances and keep the starlight fixed on the same place on the detector consistently over time.”
The ASTERIA system proved highly successful, making observations comparable to a 2-m ground-based telescope despite its only 6-cm-wide mirror. In 2020, the mission published a transit detection of an exoplanet twice as large as Earth, called 55 Cancri e, making it the first cubesat mission to detect an exoplanet. However, even by the team’s own admission, it was a marginal result.
To further improve exoplanet detections and implement systems like ASTERIA and DeMi on larger space telescopes, more tests are needed. This is precisely what has been prescribed for the next decade by Astro2020, a US National Academies-led 10-year review of the field that sets the tone for future research by identifying community priorities and recommending pathways for the next great observatories. Past decadal surveys have kickstarted the Hubble Space Telescope and the James Webb Space Telescope.
Technology demonstrations in space are essential as Sylvestre Lacour, an astrophysicist at the Paris Observatory, can attest. In 2018, Lacour and his team launched Picsat, one of the first exoplanet-dedicated cubesats that hoped to catch the possible transit of the exoplanet Beta Pictoris b. Unfortunately, its pointing system—tested on Earth but never flown in space—malfunctioned. Still, Picsat showed success in its other hardware and provided a valuable lesson on the importance of testing technology in space for the cubesat community. The mission also proved that a quick, three-year turnaround from idea to launch is possible, which could be beneficial for future, specific science projects where data is needed on a short timescale.
With all the cubesat technology successes and failures, researchers are coming closer to making cubesats that could independently conduct exoplanet research or provide essential support to larger space-based observing missions. For example, some have proposed that cubesats be dedicated to watch specific stars over long periods—something which is too expensive for major missions and too interrupted by day-night cycles for ground-based observatories.
“You could have a smaller [cubesat] mission observing a target to make sure that the time of the transit is very well known,” Lacour said. “And then the big mission could just focus on observing during the transit.”
Supporting role cubesats, solo or in fleets, could also be used to better characterize stars that exoplanets orbit and thus make inferences about the planets’ habitability, or provide additional information on the exoplanets themselves. Already cubesat missions are in progress in this regard. In September 2021, the Colorado Ultraviolet Transit Experiment (CUTE) cubesat launched to study how exoplanets can lose their atmospheres.
Some researchers have also proposed using cubesats as star shades. In direct imaging, a physical blocker is used to hide the light of the host star to reveal the much dimmer orbiting exoplanets. This is typically done within observatories using a coronagraph. However, ideas have been floated that space telescopes could employ a large shield flown hundreds of miles in front of the primary mirror. Such star shades enable better optics but would be unwieldy and cost valuable time and fuel. A fleet of cubesats might do the job instead if they can be strategically deployed around the main telescope.
While cubesats will never replace full-size space telescopes, their versatility almost ensures they will be key players in the future of exoplanet research. Whether it is as dedicated observers, platforms for testing, or allies for larger telescopes, cubesats are playing an important role in bringing us closer to finding the next Earth.
“Cubesats will always be a niche, but for sure there’s a future to this kind of project,” says Lacour.
Mara Johnson-Groh is a freelance science writer and photographer who writes about everything under the sun, and even things beyond it.
Enjoy this article? Get similar news in your inbox |
|