Nuclear fusion: NIF’s hall of mirrors may solve world’s energy crisis
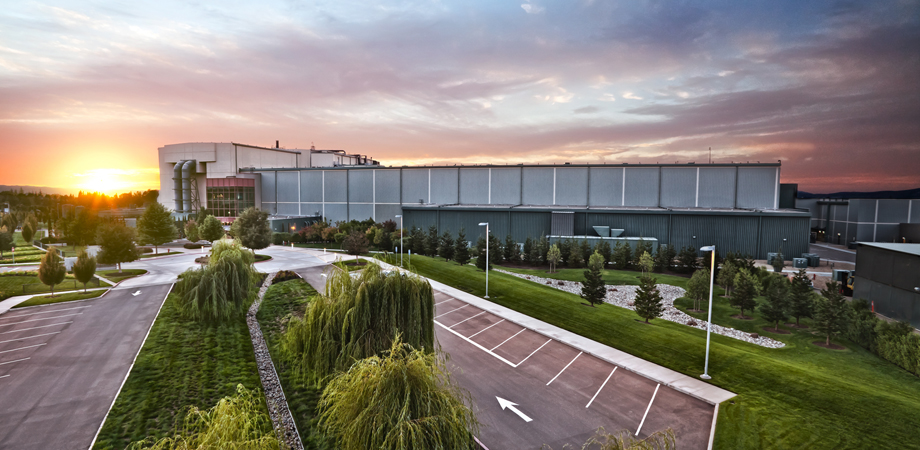
The elusive goal of nuclear fusion has represented the great hope for the world's energy future ever since physicists began seriously pursuing it in the 1950s. Fusing atoms together rather than splitting them apart, as today's fission reactors do, portends relatively limitless, safe, environmentally friendly energy. No less a mind than Stephen Hawking once said, "I would like nuclear fusion to become a practical power source. It would provide an inexhaustible supply of energy, without pollution or global warming."
But the rap on fusion: it's been 30 years in the future for over 60 years now.
There are dozens or more of ongoing initiatives, some state funded, some privately backed. Many of them — including one at the US government's National Ignition Facility (NIF) that uses 192 lasers to pull off the feat — have successfully fused different forms of hydrogen. But the underlying challenge remains: they have all put more energy into the process than the amount that has come out. None have achieved break even, known in the business as a gain of one. That's a huge hurdle, considering that experts believe that the energy out will have to be not "one," but, rather, somewhere on the order of 100 to 200 times the energy-in for fusion to be economically viable. So the days when a fusion reactor might be tied into the electricity grid feeding your home or office could still be a long way off.
For now, the next milestone would be "one."
And on that front, NIF made a great stride last August when it coaxed more energy out of its massive 192-laser apparatus than it — or, according to NIF, anyone — ever had before. Its 1.35 megajoule output was about 71% of the 1.9 megajoules that it poured in. While 71% — 0.71 — is still a fair distance from one, it was a huge advance over the 9% that was NIF's previous best.
Improvements in optics
At SPIE Photonics West in January, NIF's Tammy Ma delivered a plenary presentation describing the improvements in optics that helped to achieve the stunning leap to 71%. She also outlined the emerging optical breakthroughs that should help her and her fellow physicists reach "one" and beyond.
NIF physicist Dr. Tammy Ma. Credit: NIF/Lawrence Livermore National Laboratory
First, a quick primer on how the apparatus works at NIF, a giant facility which is 10 stories tall and covers an area the equivalent of three football fields. Like many fusion projects, NIF is combining isotopes of hydrogen known as deuterium and tritium. Synthesizing those two into one entity yields helium, a neutron, and — crucially — heat. In most fusion projects, the heat is the energy out that would drive a turbine or deliver heat for other purposes such as industrial processes. (Another approach to fusion, called aneutronic, actually delivers electricity directly, cutting out the turbine). Historically, there are two methods for mating deuterium and tritium, although other approaches have emerged over the last couple of decades.
NIF uses the newer of the two, called "inertial confinement." It places the two isotopes in a tiny BB-sized pellet, packed to 100 times the density of lead. NIF fires 192 lasers at this target, with each laser traveling a mile in a back-and-forth pattern, passing through slabs of optical glass that boost the laser's power by over quadrillion times on its way to the target. The combination of the high powered lasers and the intensely confined space triggers temperatures that flash to as high as 100 degrees C, prompting the isotopes to fuse. The fusion happens in perhaps less than a billionth of a second.
Inertial confinement contrasts with the original idea for a fusion machine, called a tokamak. There are probably scores of experimental tokamaks around the world. The technology is still in development today and is epitomized by the 100-foot tall, 830-cubic meter tokamak under construction in Cadarache, France, backed by the EU and by six member nations China, Russia, Japan, South Korea, and the US; a total of 35 nations are involved, including non-members. In the tokamak design, a sparse deuterium-tritium plasma roams a comparatively big but magnetically confined space and is super heated until fusion happens.
This cylinder, called a hohlraum, contains the BB-sized (~5mm) fuel capsule. Credit: NIF/Lawrence Livermore National Laboratory
So how did NIF get to its best ever 1.35 megajoules in August?
Ma notes that while improved optics played a role, much of the boost came from significant refinements in the design of the target pellet, which was smoother in material and more densely packed than for any previous NIF shot.
Optically, it didn't hurt that NIF had improved its master oscillator room — the originator of a single relatively low power laser that becomes 192 separate and extraordinarily more powerful lasers. It also managed to attain an exceptionally good power balance and power accuracy across the 192 lasers — sometimes, the performance of a laser can stray from what the engineers prescribe for each one in a shot, but on this occasion, as Ma notes, they behaved well.
Laser amplification
Ongoing refurbishment of the slabs of thick optical glass that amplify the lasers' power certainly helped. NIF shoots its lasers every 4 to 8 hours not only for fusion energy experiments but for other purposes as well such as nuclear weapons research and studying planets, stars and the universe. The 40cm x 40cm glass, which is a few inches thick, can take a beating. In the amplification process, first the glass is energized, and then the glass conveys that energy to the laser passing through it. Damage incurred during that process can grow and throw off the accuracy of a laser in subsequent shots.
"We run the lasers right at the hairy edge of what those optics can actually handle," says Ma. Thus, NIF routinely pulls out the glass slabs for the purpose of examining them and polishing them.
The lasers converge at this point as they near the target. Credit: NIF/Lawrence Livermore National Laboratory
The amplifiers are one example of some 7500 large aperture optics that the lasers pass through. The place is awash with optics; maintenance is critical. But the time taken for upkeep, as well as the limitations of the glass, are factors in both curtailing the number of shots and in undermining both the power levels and efficiency of them.
That's where NIF is eyeing improvements. Glass amplifiers that can handle higher powers and that can more efficiently boost laser power, should ultimately make better use of the "energy-in," and thus lead to a better energy-out ratio.
Improving the optics
Ma, who is NIF's program element leader for high intensity laser science, has identified several technologies that could improve the optics.
One method involves shortening the amount of time it takes for the glass to cool down after a shot. Prolonged cooling today is one of the main reasons why shots can't happen more often than every four to eight hours. With NIF examining the possibility of moving to lasers that can fire at 10 Hertz — 10 times a second — it will need glass that is easier to cool. Hope lies in the form of chopping the optics into strips and running cooling gas between the strips.
That's one idea.
Another entails upgrading the material used for optical glass so that it is more effective at gathering a laser's photons and energizing them. Ma is hopeful that new "gain media," such as thulium-doped, yttrium lithium fluoride, will make a difference.
Likewise, improvements are underway in the lasers used to energize the glass in the first place. Today's glass take its energy from flashes of white laser light. That's a highly inefficient process, because the glass absorbs only certain wavelengths out of the white. As Ma notes, "you are wasting every other color of the rainbow except the one's that useful to you."
The flash light is a double whammy, in that it also gives off excess heat, requiring energy-consuming cooling systems to eliminate.
The solution? Color laser diode pumps that emit only the useful color would eliminate the waste, and would run at cooler temperatures.
Maintenance and reuse of optics is critical to precision operations at NIF. Credit: NIF/Lawrence Livermore National Laboratory.
Work is underway in all areas at various institutions. There is plenty of promise and potential, and also some concerns. For instance, color laser diode pumps of the magnitude needed for the optical glass in fusion is much more expensive than the flash lamps used today.
In fact, all these improvements would come at a certain monetary cost. In the near future, NIF is not planning on using any of them.
"We're not tearing things out," says Ma. Rather it will concentrate on improving the maintenance, and on the hardening of existing optics. That alone should help NIF to achieve an input power of 2.6 megajoules and to get to a gain of one within a few years, in Ma's estimation.
But the new stuff, says Ma, will have to wait. In fact, Ma envisions it not going into the existing NIF, which opened in 2009 at a cost approach $4 billion, after a decade or so of construction and delays. Rather, she says, it's time for a new NIF.
Mark Halper is a freelance business, technology, and science journalist who covers everything from media moguls to subatomic particles. A version of this article was originally published in the 2022 Photonics West Show Daily.
Enjoy this article? Get similar news in your inbox |
|