Engineers Search for a Tiny Cure for a Big Problem
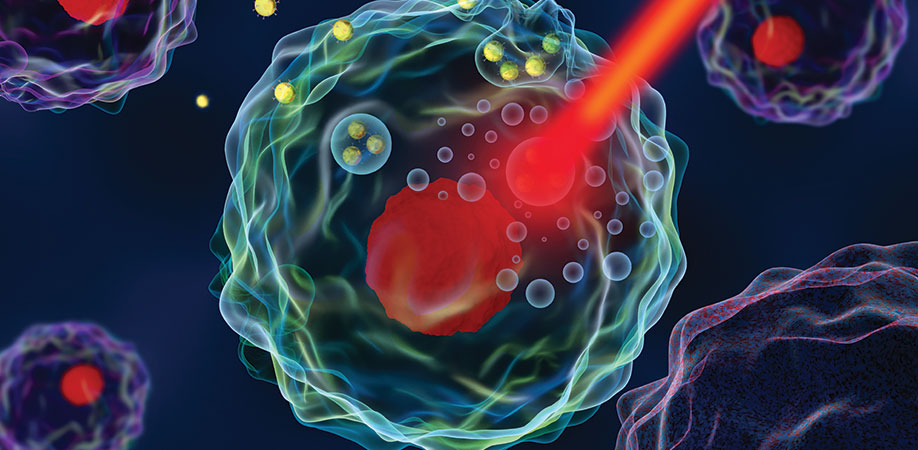
Prostate screening is a routine step of the annual checkup for most men over age 54. The test checks for prostate-specific antigen (PSA), where anything over 4 is a concern, and anything over 10 is bad news. In 2018, Frank Billingsley, a popular meteorologist in Houston, Texas, learned during his annual checkup that his PSA was 25.
He was lucky on two counts: first, the cancer hadn't spread to his bone, and second, his public persona brought his case to the attention of Rice University, where a team of researchers was working to develop a highly targeted treatment for prostate cancer using gold nanoparticles. And their treatment had just been cleared by the FDA for clinical trial.
Billingsley underwent the treatment in November 2018, and a week later, tests showed that the cancer was gone. The study leads, Naomi Halas of Rice University and Jennifer West of Duke University, were ecstatic. "We had the first, really engineered nanoparticle to go into human beings," West said in a 2019 interview. "In the beginning, the FDA wasn't sure how to handle these types of materials. We had something that looked like an injectable liquid in an IV bag. Was it a drug or a device?"
The FDA's confusion is not surprising, and it is just one of many hurdles that this technology has encountered over the years. Nanoparticles have actually been a golden child of cancer research for a couple of decades, but for an idea that makes such perfect theoretical sense, it has run into a lot of practical problems.
Leaky Vasculature
Research into nanoparticles as a cancer therapeutic goes back to the 1970s. Doxorubicin—originally made from the bacteria Streptomyces peucetius—is one of the oldest successful cancer treatment drugs on the market. In an attempt to improve its efficacy, researchers tried encapsulating the drug into a liposome, which created tiny little drug droplets. To their surprise, these nanosized drug particles accumulated more in tumors than the free drug.
This phenomenon is due to a peculiar trait known as the enhanced permission and retention (EPR) effect, and more whimsically called "leaky vasculature." Unlike healthy tissue, the vessels that supply blood to tumors have fairly large perforations. Nanoparticles can pass harmlessly by normal tissue, and then fall through the leaky pipes into the tumor. Because tumors also have a deficient drainage system, the nanoparticles aren't easily flushed out.
Once the EPR effect was understood by researchers, the possibilities of nanoparticles for cancer treatment exploded in scientists' imaginations. Imagine a magic bullet that could target only the rogue cancer cells and ignore healthy cells. The harsh physical effects of chemotherapy and radiation-nausea, weight loss, weakness, hair loss-could be eliminated.
But, as with most medical discoveries, figuring out the magic has been harder than designing the bullet. First, nanoparticles are foreign objects, so as soon as the body detects them, it wants to flush them out via the liver, kidneys, or spleen. The opposite is true for cancer cells, which are the body's own cells, so the immune system wants to protect them. Also, it turns out that there's a lot of variation in tumors, even within one patient. Some are poorly vasculated, and some don't have leaky vasculature at all, which makes them inaccessible to nanomedicine. And even when nanomedicine works perfectly in mice models—a necessary prerequisite for regulatory approval of any medical treatment—for some reason it often doesn't work the same way in humans.
To better understand how these nanoparticles are getting to—or not getting to—a tumor site, some research groups pivoted from using nanoparticles to treat cancer, to using nanoparticles to better visualize the drug's arrival at the cancer site, a field known as image-guided therapy.
Illustration of phase-changing nanodroplets, activated by a light trigger. Credit: Catalina-Paula Spatarelu
Catalina-Paula Spatarelu is a graduate research assistant in the Thayer School of Engineering at Dartmouth. She wants to increase the likelihood that the drugs get to the cancer site without affecting other organs in the body. And then she wants visual confirmation that they got there. To accomplish this feat, she designed a nanodroplet with as many functions as a Swiss Army knife.
First and foremost, the nanodroplet carries two well-known cancer drugs. The core of the particle contains the cancer drug cisplatin, which is emulsified inside of a liquid called perfluorocarbon. Surrounding that is a lipid shell that holds the whole thing together, which incorporates both paclitaxel, the second cancer drug, and a specific photosensitive dye.
These nanodroplets are injected into the bloodstream near the tumor site, and then activated with a near-infrared light. That light excites the dye embedded in the lipid shell, which starts vibrating and generates heat. This causes the perfluorocarbon in the core to turn from liquid to gas, which creates a bubble.
When the droplet transitions into a bubble, three important things happen: First, the movement of those microbubbles helps enlarge the permeations in the blood vessels so that more of the drug gets into the tumor. Second, the two drugs it contained are released. And third, the bubbles create a contrast for ultrasound, which makes the whole process visible using a very standard clinical tool, and allows researchers to visually confirm that the drug was delivered where they intended.
Spatarelu thinks the nanodroplet system has a lot of promise. "We're trying to avoid having drugs spread everywhere in the body, because with systematic chemo therapeutics, it can't distinguish between cancer tissue and normal tissue. So you're limited in how much you can use," she says. "But if we can get more of the drug in the tumor, then we can potentially increase that dose and be more aggressive when we need to."
Spatarelu likes to remind people that cancer cells are delicate things. They can be killed by simply overhydrating them in a petri dish—and they can also be killed by heat. While she is looking to nanodroplets to deliver drugs to a tumor site, other researchers are looking for ways to cook the little critters. A mere 43°C (109°F) will kill a cancer cell. Unfortunately, that temp is also high enough to damage healthy cells.
But what if the cancer cells could be heated without impacting the neighboring tissue? There are two types of metal nanoparticles that hold some promise. The first is superparamagnetic iron oxide nanoparticles (SPIONs). As you might guess from the name, they are super magnetic. They also have the benefit of being made from an element that the body needs—iron—as opposed to some other nanomaterials that have low cytotoxic effects. Researchers have learned that if they can get those SPIONs to the tumor site, then a magnetic field can harmlessly penetrate the body and activate those SPIONs, which causes them to vibrate and heat up, killing the cells that they're attached to.
Gold nanoparticles of the sort used in the successful clinical trial for prostate cancer work via a similar principle. But instead of being activated by a magnetic field, gold nanoparticles are activated by near-infrared light, which can penetrate deep into the body, and can be directed and localized a bit better than a magnetic field. As the gold nanoparticles absorb the light, they convert it to heat, induce hyperthermia, and destroy the tumor cells that they are adjacent to.
The Journey from Lab to Clinic
We've just covered three approaches to cancer treatment, all of them utilizing the EPR effect to deliver small nanoparticles to the cancer site—and one of them has even shown early clinical success. So surely a nanocure for other types of cancer, in addition to prostate, is around the corner?
"Well, the EPR effect happens to work quite well in mice, but when researchers try to transition to people it hasn't worked as well," explains Geoff Luke, Spatarelu's lab supervisor at Dartmouth. His comment helps explain why the success of Halas's gold nanoparticle clinical trial is so remarkable. Researchers have successfully used nanoparticles to kill cancer cells in mice over and over again, but when they move onto human cells, it often doesn't work.
Halas attributes their successful transition from lab to clinic to very careful background work and preparation, including development of a near-infrared light delivery system that can access the tumor and years of toxicity studies to establish safety of nanoparticles. She says, "Every step was fulfilled and fit together extremely well. It is not a ‘step' from the lab to the clinic, it is a journey, and it can be a treacherous one if a step is missing, and an utter failure if several steps are missing or fail."
Luke and Spatarelu are just beginning that journey by testing the nanodroplet drug delivery system in mice. "Each mouse has two different tumors on it. All the nanoparticles go everywhere," explains Luke. "They go to both tumors, but we only activate the droplets to make the tumor more permeable on one side. And then we hope to see that we do indeed get significantly more [drug] accumulation in that one side."
But if most researchers aren't seeing a good translation from mouse studies to humans, then are Luke and Spatarelu confident that the nanodroplets will work? "That's always a question," says Spatarelu. "But all tests that can be clinically tested in human trials have to go through the mouse process."
And there's the rub.
Changing the Formula of the Regulatory Process
Halima Alem, associate professor of physical chemistry at Lorraine University and junior member of the Institut Universitaire de France, is primarily working with the magnetic SPIONs approach to theranostics, and she is concerned by this catch-22. "Only maybe 8 percent of animal tests that give positive results can be transferred to humans. That means 92 percent of animals are sacrificed for nothing," she says.
Rather than feel frustrated about the problem, she's investigating a way to test nanomaterials directly on human cells, and cut the animal tests out of the equation. To do that, she's building human organs on a chip.
Organs-on-chip (OOC) attempt to recreate the functionality of the human physiological response outside of the human body, and on a millimeter scale. They are real human cells grown on a small plastic slide, connected by microfluidic networks that mimic vasculature. "With organ-on-chip systems you can completely mimic the patient. You can see if the drug will work on the patient in advance. But you can also understand why a drug does not work," says Alem.
SEM image showing human ciliated cells inside Emulate's lung-chip. Credit: Emulate
One of many issues with treating cancer is that people have such unique physiology. A nanotherapy that works on patient A doesn't work on patient B. Variables like blood pressure, which can vary from hour to hour even in the same person, can have a mechanical impact on movement of nanoparticles. A person's blood type and the amount of protein in the blood can also have an impact on nanoparticles. "Humans are almost the same, but when you look at the very bottom level, we can be very different. And those differences can influence the drug efficacy," says Alem.
Alem wants to design a chip that takes into account the entire journey of the nanoparticles, including leaky vasculature, healthy tissue and cancer tissue, and even the microphages that work against cancer researchers by doing their biological job to clear the foreign nanoparticles out of the system.
If OOC gain traction as a legitimate alternative—or even superior approach—to animal testing, the impact could be profound. Alem points out that the regulatory process is currently based on animal data, but "if organs-on-chip could convince the authorities, you will save a lot of time and resources without sacrificing animals. You could go directly from the drug and chip to humans and save a lot of time. You can also test different drugs in one chip without harming the patient."
A few pharmaceutical companies, like Roche Pharma, Merck, and Janssen Biotech, a research division of Johnson & Johnson, are exploring the possibilities of OOC for drug discovery. Biotech company Emulate, Inc., is supplying these companies with intestine-chips, lung-chips, and others to help them predict the potential human response of drugs. While this process can't yet replace animal models in FDA regulatory approval for drug therapy (that machinery moves slowly), it does help eliminate some of the guesswork, and ensure that the drugs that do make it to animal testing have a much higher chance of human efficacy.
Nonetheless, the FDA is paying attention to OOC. In 2017, Emulate announced a "cooperative research and development agreement" with the US FDA, during which the FDA's Office of Foods and Veterinary Medicine will evaluate OOC as a platform for toxicology testing in the areas of foods, dietary supplements, and cosmetics. That's still a big step away from replacing animal testing for pharmaceuticals, but even cautious interest from the FDA could be considered a step in the right direction.
For many people doing cancer research, personalized medicine is the holy grail. Physicians want to be able to understand what went wrong to cause the tumor in the first place, pinpoint all of the cancerous cells, and fix it using exactly the right drugs delivered to only those cells. A one-two punch of nanoparticles and OOC could hold promise for this vision.
OOC could allow physicians to create personalized chips from patients' own cells, test drugs on the chip, and determine the patient's individual response to the treatment before a drug is even administered. In combination with targeted nanotherapy—whether it's gold NP, SPIONs, nanodroplets, or one of many other nanotherapies under investigation—the magic bullet of personalized medicine for cancer therapy may someday be ready to fire.
Gwen Weerts is the managing editor of Photonics Focus.
Enjoy this article? Get similar news in your inbox |
|